Molecular clock could greatly improve smartphone navigation
Molecular clock could greatly improve smartphone navigation
Novel chip keeps time using the constant, measurable rotation of molecules as a timing reference.
MIT researchers have developed the first molecular clock on a chip, which uses the constant, measurable rotation of molecules — when exposed to a certain frequency of electromagnetic radiation — to keep time. The chip could one day significantly improve the accuracy and performance of navigation on smartphones and other consumer devices.
Today’s most accurate time-keepers are atomic clocks. These clocks rely on the steady resonance of atoms, when exposed to a specific frequency, to measure exactly one second. Several such clocks are installed in all GPS satellites. By “trilaterating” time signals broadcast from these satellites — a technique like triangulation, that uses 3-D dimensional data for positioning — your smartphone and other ground receivers can pinpoint their own location.
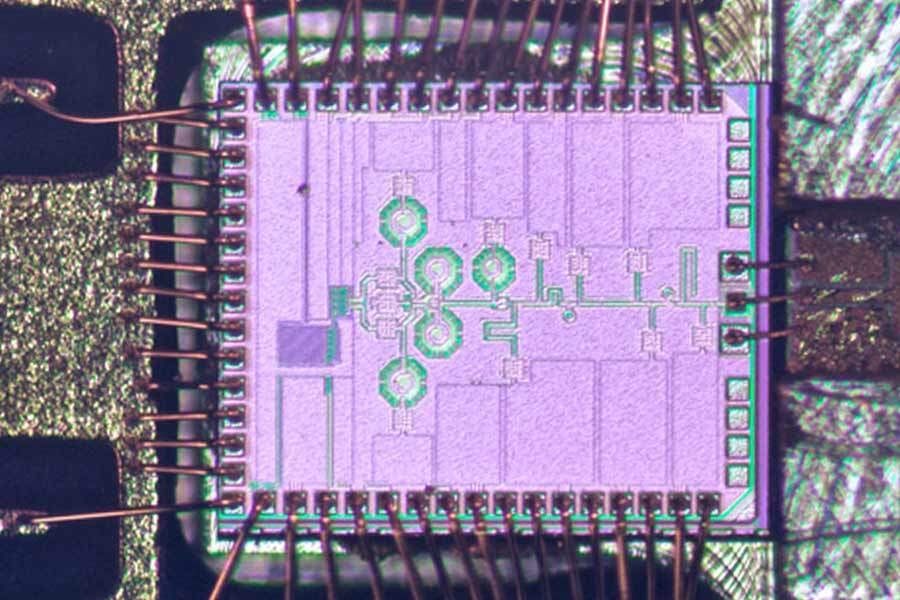
The clock transmitter chip (pink) wired to a circuit board package. Connected is a metal gas cell (right), in which a 231.061 GHz signal generated from the chip excites the rotation of carbonyl sulfide molecules. Because the peak rotation of the molecules is constant, it can be used as a reference point to keep accurate time. Courtesy of the researchers
But atomic clocks are large and expensive. Your smartphone, therefore, has a much less accurate internal clock that relies on three satellite signals to navigate and can still calculate wrong locations. Errors can be reduced with corrections from additional satellite signals, if available, but this degrades the performance and speed of your navigation. When signals drop or weaken — such as in areas surrounded by signal-reflecting buildings or in tunnels — your phone primarily relies on its clock and an accelerometer to estimate your location and where you’re going.
Researchers from MIT’s Department of Electrical Engineering and Computer Science (EECS) and Terahertz Integrated Electronics Group have now built an on-chip clock that exposes specific molecules — not atoms — to an exact, ultrahigh frequency that causes them to spin. When the molecular rotations cause maximum energy absorption, a periodic output is clocked — in this case, a second. As with the resonance of atoms, this spin is reliably constant enough that it can serve as a precise timing reference.
In experiments, the molecular clock averaged an error under 1 microsecond per hour, comparable to miniature atomic clocks and 10,000 times more stable than the crystal-oscillator clocks in smartphones. Because the molecular clock is fully electronic and doesn’t require bulky, power-hungry components used to insulate and excite the atoms, it is manufactured with the low-cost, complementary metal-oxide-semiconductor (CMOS) integrated circuit technology used to make all smartphone chips.
“Our vision is, in the future, you don’t need to spend a big chunk of money getting atomic clocks in most equipment. Rather, you just have a little gas cell that you attached to the corner of a chip in a smartphone, and then the whole thing is running at atomic clock-grade accuracy,” says Ruonan Han, an associate professor in EECS and co-author of a paper describing the molecular clock, published today in Nature Electronics.
The chip-scale molecular clock can also be used for more efficient time-keeping in operations that require location precision but involve little to no GPS signal, such as underwater sensing or battlefield applications.
Joining Han on the paper are: Cheng Wang, a PhD student and first author; Xiang Yi, a postdoc; and graduate students James Mawdsley, Mina Kim, and Zihan Wang, all from EECS.
In the 1960s, scientists officially defined one second as 9,192,631,770 oscillations of radiation, which is the exact frequency it takes for cesium-133 atoms to change from a low state to high state of excitability. Because that change is constant, that exact frequency can be used as a reliable time reference of one second. Essentially, every time 9,192,631,770 oscillations occur, one second has passed.
Atomic clocks are systems that use that concept. They sweep a narrow band of microwave frequencies across cesium-133 atoms until a maximum number of the atoms transition to their high states — meaning the frequency is then at exactly 9,192,631,770 oscillations. When that happens, the system clocks a second. It continuously tests that a maximum number of those atoms are in high-energy states and, if not, adjusts the frequency to keep on track. The best atomic clocks come within one second of error every 1.4 million years.
In recent years, the U.S. Defense Advanced Research Projects Agency has introduced chip-scale atomic clocks. But these run about $1,000 each — too pricey for consumer devices. To shrink the scale, “we searched for different physics all together,” Han says. “We don’t probe the behavior of atoms; rather, we probe the behavior of molecules.”
The researchers’ chip functions similarly to an atomic clock but relies on measuring the rotation of the molecule carbonyl sulfide (OCS), when exposed to certain frequencies. Attached to the chip is a gas cell filled with OCS. A circuit continuously sweeps frequencies of electromagnetic waves along the cell, causing the molecules to start rotating. A receiver measures the energy of these rotations and adjusts the clock output frequency accordingly. At a frequency very close to 231.060983 gigahertz, the molecules reach peak rotation and form a sharp signal response. The researchers divided down that frequency to exactly one second, matching it with the official time from atomic clocks.
“The output of the system is linked to that known number — about 231 gigahertz,” Han says. “You want to correlate a quantity that is useful to you with a quantity that is physical constant, that doesn’t change. Then your quantity becomes very stable.”
A key challenge was designing a chip that can shoot out a 200-gigahertz signal to make a molecule rotate. Consumer device components can generally only produce a few gigahertz of signal strength. The researchers developed custom metal structures and other components that increase the efficacy of transistors, in order to shape a low-frequency input signal into a higher-frequency electromagnetic wave, while using as little power as possible. The chip consumes only 66 milliwatts of power. For comparison, common smartphone features — such as GPS, Wi-Fi, and LED lighting —can consume hundreds of milliwatts during use.
The chips could be used for underwater sensing, where GPS signals aren’t available, Han says. In those applications, sonic waves are shot into the ocean floor and return to a grid of underwater sensors. Inside each sensor, an attached atomic clock measures the signal delay to pinpoint the location of, say, oil under the ocean floor. The researchers’ chip could be a low-power and low-cost alternative to the atomic clocks.
The chip could also be used on the battlefield, Han says. Bombs are often remotely triggered on battlefields, so soldiers use equipment that suppresses all signals in the area so the bombs won’t go off. “Soldiers themselves then don’t have GPS signals anymore,” Han says. “Those are places when an accurate internal clock for local navigation becomes quite essential.”
Currently, the prototype needs some fine-tuning before it’s ready to reach consumer devices. The researchers currently have plans to shrink the clock even more and reduce the average power consumption to a few milliwatts, while cutting its error rate by another one or two orders of magnitude.
This work was supported by a National Science Foundation CAREER award, MIT Lincoln Laboratory, MIT Center of Integrated Circuits and Systems, and a Texas Instruments Fellowship.