Coral-Shaped Nanoparticles Built Using Engineered Peptoids
Coral-shaped nanoparticles built by design using engineered peptoids
Scientists manipulate shapes while enhancing optical properties of tiny particles
Coral-shaped nanoparticles discovery at
Researcher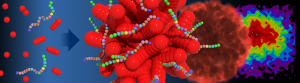
In a new study led by PNNL materials scientist Chun-Long Chen, sequence-defined peptoids (shown here as chains of small colorful spheres) enabled the predictive morphologic evolution of spherical coral-shaped gold nanoparticles (middle). The gold nanoparticles exhibited a plasmonic enhancement as high as 105 fold (right).
s have long worked to address a grand challenge in synthesis science: to design and synthesize bio-inspired functional materials that rival those found in biology. If we can learn how to mimic in vivo functions of natural proteins—such as how biomolecules (e.g., proteins) and inorganic salts interact to form teeth, bones, or shell minerals—researchers might be able to apply the discovery to produce highly complex, designable, hybrid materials for energy-related applications.
In a paper recently published in Nature Communications, Chun-Long Chen, a materials scientist at Pacific Northwest National Laboratory, described how his multidisciplinary team sought to understand and harness the complex functionality of hierarchical matter and predict its shape and function. The team carefully designed peptoids, types of sequence-defined synthetic molecules, to control the formation of spherical coral-shaped gold nanoparticles. They also sought to understand how the peptoid molecule functions during particle formation, how peptoids interact with each other, and how they bind to gold’s surface. Along the way, they discovered how these mechanistic studies can write the rules for designing peptoids for predictive materials synthesis.
“The process was fascinating,” said Chen. “Controlling the nucleation, growth kinetics, and morphology of nanostructured inorganic materials with sequence-defined molecules to intentionally produce these spherical coral-shaped nanostructures gave us confidence that other shapes could be achieved using similar methods.”
Their work led to an important feat in materials synthesis: the development of a rule-of-thumb for designing peptoids that enable predictive synthesis of coral-shaped gold nanoparticles. These individual gold particles exhibit a plasmonic enhancement as high as 105 fold.
Why it matters: While natural organisms make a wide variety of exquisitely complex, nano-, micro-, and macroscale functional materials at high yields in an energy-efficient and highly reproducible manner, all under rather mild aqueous synthetic conditions, achieving such precise control over nanoparticle morphology is challenging. Being able to do so is important to future technological applications of nanoparticles. The level of control and complexity of the nanostructures in this particular study stand out and, as Chen notes, pushes us closer to predictive, bio-inspired, materials synthesis.
The team’s peptoid-based, biomimetric approach generates complex, functional nanomaterials under mild aqueous synthetic conditions; researchers studying the synthesis of hybrid materials for energy-related applications (e.g., solar energy or battery applications) would likely find them of interest.
Summary: Achieving the predictable synthesis of inorganic nanomaterials is a long-standing challenge. If scientists can develop the rules for precisely controlling materials morphology—as Nature does during biomineral formation—they can use these bio-inspired approaches to produce highly complex, designable, hybrid materials in a rational or even predictable way for energy-related applications.
In the past, many researchers have used biomolecules—particularly proteins and peptides—to develop ways to control the formation of nanomaterials, but the rules for designing molecules that lead to the formation of materials with predictable morphologies are still unknown. In this study, Chen and his team decided to use peptoids to control the formation of gold nanomaterials. They specifically used peptoids (instead of proteins and peptides) for three reasons: peptoids do not have the intrinsic complexity caused by backbone folding, peptoids have similar or even larger variations of side chains, and peptoids have higher chemical and thermal stabilities.
The team imagined the high-information content of peptoid molecules would give them control over the materials formation and would reveal the rules behind the formation process.
“The success of this research is a beautiful example of teamwork,” said Chen.
The team’s paper, published in Nature Communications as “Controlled synthesis of highly-branched plasmonic gold nanoparticles through peptoid engineering,” describes that teamwork in detail. Steps included designing and synthesizing peptoids with rational modifications of their chemistries; using state-of-art fluid cell transmission electron microscopy (TEM) to observe how the particles form, attach, and merge together into clusters of nanorods in real time; using molecular dynamics simulations to show how peptoid molecules with variable chemistries interact with gold; and using time-of-flight secondary ion mass spectrometry and X-ray photoelectron spectroscopy techniques to experimentally confirm the computational predictions.
Through these mechanistic studies, the team gained a clear understanding of peptoid-controlled coral-shaped gold nanoparticle formation. This helped them to develop a rule of thumb for designing peptoids that predictively enabled the morphological evolution from spherical to coral-shaped nanoparticles. The team also found that the individual coral-shaped gold nanoparticles displayed a plasmonic enhancement as high as 105 fold, and they were able to expand this peptoid-based approach for controlled synthesis of other coral-shaped nanoparticles, highlighting its broad utility.
What’s next? Achieving a high level of regulation over morphology—seen when biomineral formation is controlled by proteins and peptides—is still a significant challenge and the rules governing bio-controlled formation of nanomaterials remain unknown. Building on their success discerning the rules for making coral-shaped nanoparticles, Chen and his team have new shapes in mind. They are currently working toward achieving a similar understanding of the peptoid-controlled formation of other morphologies, including five-pointed star-shaped gold nanoparticles and sheet-like silver nanoparticle assemblies. He expects this peptoid-based approach could lead to the creation of a wide range of complex morphologies and will eventually be useful for developing a predictive synthesis of nanomaterials that have complex morphologies and programmable functions.
PNNL’s focus on materials science was enhanced this year when the Lab formalized a partnership with the University of Washington to launch the Northwest Institute for Materials Physics, Chemistry, and Technology (NW Impact). The two institutions will share resources, instruments, and opportunities as they work to accelerate transformative advances in technology through the science of making materials to drive discoveries and innovation in energy, telecommunications, medicine, information technology, and other fields.
Acknowledgments
This work is related to the Basic Research Needs for Synthesis Science for Energy Relevant Technology, specifically the following Priority Research Directions: 1) harness the complex functionality of hierarchical matter, and 2) accelerate materials discovery by exploiting extreme conditions, complex chemistries and molecules, and interfacial systems.
Sponsors: This work was primarily supported by the Materials Synthesis and Simulation Across Scales (MS3) Initiative through the Laboratory Directed Research and Development (LDRD) program at PNNL. The Department of Energy’s Office of Science, Office of Basic Energy Sciences supported the peptoid synthesis as part of the Biomolecular Materials Program at PNNL. In situ TEM studies were supported by a Doctoral Dissertation Fellowship to Lili Liu from Texas Tech University. Access to computational resources at the National Computing Infrastructure and the Pawsey Supercomputing Centre was made possible in part by funding from the Australian Government and the Government of Western Australia.
Research Area: Materials Synthesis and Simulation Across Scales Initiative
User Facilities: ToF-SIMS measurements were performed using the W. R. Wiley Environmental Molecular Sciences Laboratory (EMSL), a national scientific user facility sponsored by the Department of Energy’s Office of Biological and Environmental Research and located at Pacific Northwest National Laboratory.
Research Team: Chun-Long Chen, Yu Gong, Patrick Z. El-Khoury, Lili Liu, Feng Yan, and Xin Zhang (Pacific Northwest National Laboratory); Mark H. Engelhard, Yanyan Zhang, and Zihua Zhu (Environmental Molecular Sciences Laboratory at PNNL); James J. DeYoreo (Pacific Northwest National Laboratory and University of Washington); and Tiffany R. Walsh (Institute for Frontier Materials at Deakin University).
Reference: Feng Yan, Lili Liu, Tiffany R. Walsh, Yu Gong, Patrick Z. El-Khoury, Yanyan Zhang, Zihua Zhu, James J. DeYoreo, Mark H. Engelhard, Xin Zhang, Chun-Long Chen. 2018. “Controlled synthesis of highly-branched plasmonic gold nanoparticles through peptoid engineering.” Nature Communications, June 13, 2018. DOI: 10.1038/s41467-018-04789-2
Source: Pacific Northwest National Laboratory
Keywords: Coral-shaped nanoparticles, engineered peptoids, Nanoscience, Nanotechnology, Biotechnology